RESEARCH DIRECTIONS

Quantum Information Processing
Quantum processors realized with superconducting Quantum processors realized with superconducting circuits are now among the most advanced. These devices rely on transmon qubits operated in a circuit QED architecture. The ‘Theory of Superconducting Quantum Devices’ group has played a leading role in the development of these concepts. Our current focus is on designing more robust superconducting qubits including bosonic qubits, developing novel two-qubit gates with fidelity allowing for fault tolerance, and on creating quantum algorithms that are compatible with current and near-term devices.

Qubit Readout
Qubit readout in circuit QED is based on the dispersive qubit-resonator interaction. First proposed in 2004, this approach is now routinely used in laboratories worldwide to realize high-fidelity single-shot qubit readout. The group is also developing microwave components, such as quantum-limited amplifiers and circulators, necessary to increase the fidelity of the readout process. We have also developed novel approaches to qubit readout, such as the longitudinal readout, and approaches to measure qubit parity for quantum error correction.

Quantum Optics
Circuit QED is a realization of the physics of cavity QED in novel parameter regimes. Thanks to the flexibility of superconducting quantum devices, it is possible to obtain significantly larger light-matter coupling in circuit QED than in cavity QED. Moreover, strong nonlinearity at the single photon level is easily realized. Thanks to this, circuit QED is an ideal playground to study the rich physics of quantum optics on a chip. In this context, the ‘Theory of Superconducting Quantum Devices’ group has shown how to reach the ultrastrong coupling regime with superconducting qubits. We have introduced single microwave photon detectors, with application to the search for dark matter, and have contributed to the firsts tests of classic predictions of quantum optics now made possible in circuit QED.

Hybrid Quantum Systems
Hybrid quantum systems consist of heterogeneous physical systems that are combined to take advantage of their individual unique advantages. An example is the use of superconducting microwave resonators to mediate long-distance interaction between spin qubits realized in a semiconductor. The group has contributed to the first experiments showing the strong coupling between a single photon in a microwave resonator and a single spin in a semiconducting quantum dot, and to experiments demonstrating the coherent coupling of a semiconducting quantum dot to a superconducting qubit.
Image: P. Scarlino, D. J. van Woerkom, U. C. Mendes, J. V. Koski, A. J. Landig, C. K. Andersen, S. Gasparinetti, C. Reichl, W. Wegscheider, K. Ensslin, T. Ihn, A. Blais, A. Wallraff: Coherent microwave-photon-mediated coupling between a semiconductor and a superconducting qubit. In: Nature Communications 10 (1), 3011 (2019), ISBN: 2041-1723.
RESEARCH ENVIRONMENT
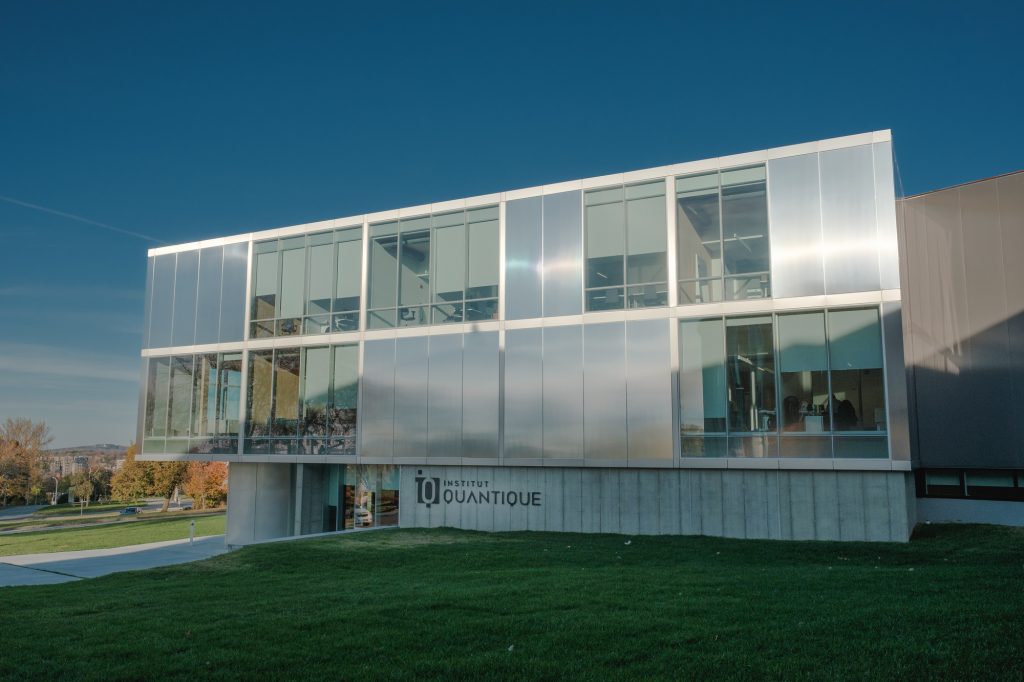
The Quantum Institute (QI) at the Université de Sherbrooke brings together experts in quantum information, quantum engineering and quantum materials to accelerate the transition from quantum science to quantum technologies. The Institute now has more than 300 members, including 250 students and postdoctoral fellows, as well as 32 professor-researchers.
The Institute’s new research building, opening in September 2021, was designed with its community at heart, with open interaction spaces and blackboard walls, state-of-the-art digital computing infrastructure, and a Quantum FabLab open to all members. The new building is a unique environment favoring collaboration, creativity and innovation.
As home to Canada’s first IBM Q Hub, IQ members have privileged access to IBM’s most advanced quantum computing systems, opening up a world of research possibilities in a unique environment where collaborative projects are developed in partnership with industry.