Nuclear Magnetic Resonance (NMR)
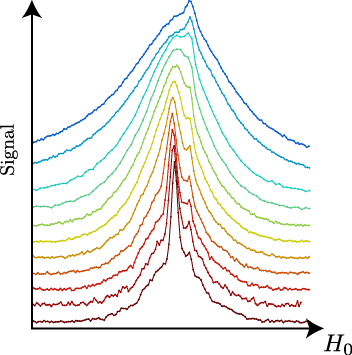
Fig. 1: 51V NMR spectra of the kagome antiferromagnet Vesignieite [1].
Solid-state NMR makes use of the nuclear spins within a material to obtain a distribution of internal magnetic fields. A large and homogeneous external magnetic field, H0, is applied to polarize the nuclear spins. Then, perpendicular field pulses are applied which cause the spins to tip and precess around the static field with a frequency f = H0γ/2π, where γ is the gyromagnetic ratio of the nucleus in question. The variations in frequency of the resulting free-induction decay (or spin echo) indicate the variations in magnetic field within a material. Furthermore, by looking at the rates at which the precession decays, the relaxation, one can determine how quickly the fields within a material are fluctuating. NMR is a very versatile tool that has applications in virtually all domains of condensed matter physics, from superconductivity to quantum magnetism.